
Titanium Anodizing Science
Titanium: A Not-so Noble Metal
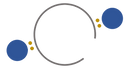
Titanium isn't corrosion resistant after all...
Few metals are actually resistant to corrosion when exposed to oxygen. But a limited group of "Noble Metals", including gold, silver, and platinum, are able to claim the elevated stature of oxidation invulnerability. Although it is often noted for its corrosion resistance, titanium is actually not a member of the noble class and will readily react with oxygen to form a thin layer of titanium dioxide (TiO2) on its surface. But your Litespeed salesperson wasn't lying to you about the lifetime of corrosion resistance. The very thin oxide layer (1-20 nm thick depending on exposure time with the atmosphere) that forms naturally provides a protective barrier against continued oxidation of the underlying Ti metal. It is actually this layer of TiO2 that has given titanium its reputation when it comes to withstanding the elements.


How Titanium Anodizing Works
How electricity and chemistry form TiO2
Titanium anodizing describes the process of using an electrolytic cell to build a specified thickness of oxide (TiO2) on the surface of titanium parts (perferably in the form of bike frames, components, or bottle cages). If you're not familiar with the parts of an electrolytic cell and how it works, then buckle-up buttercup, and check out the fairly awesome graphics below. If you are familiar... well...

In general, the anodizing setup involves connecting a titanium workpiece/part to the positive terminal of a DC power supply and submerging it in an electrolyte solution. You've now got the anode half of the party in place. A second conductive piece of metal, the cathode, is also submerged in the electrolyte solution (making sure to avoid contact with the anode!) and connected to the negative terminal of the power supply.
​
Activate the power supply and the magic of chemistry happens. The generated current and voltage differential between the anode and cathode forces the electrons within the electrolytic cell to boogie. Moving in the direction of the current, electrons are removed from electrolyte solution at the anode, and returned at the cathode. It is this transfer of electrons that drives oxidation-reduction reactions at the anode and cathode, respectively, and utlimately leads to the formation of TiO2 on the surface of the Ti anode. The oxidation and reduction reactions that are necessary for anodizing (and electrolysis in general for all you hydrogen junkies) are possible due to the asymetric molecular structure of a water. The H2O molecule is characterized by a polar covalent bond between oxygen and hydrogen atoms. The bond is relatively weak and results in an uneven charge density across the molecule. The oxygen side carries a slightly negative charge, while the hydrogen side of the molecule is slightly positive in charge. And it is this small characteristic of H2O that makes anodizing possible. Within the electrolytic cell, the negative charge of the cathode pulls at the hydrogen side of the H2O molecules, while the oxygen side is pulled toward the positive charge of the anode.
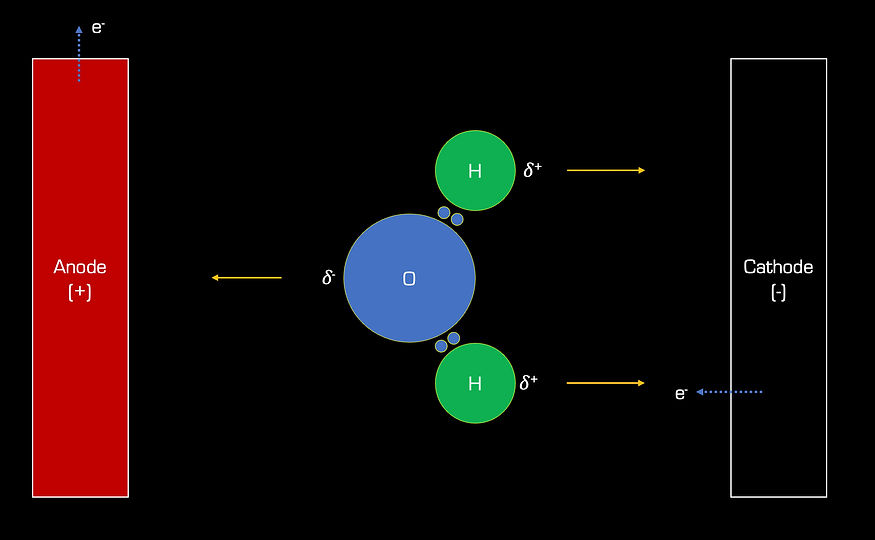
As each free electron enters the solution at the cathode, the freebie negative charge is too much for one of the H atoms of a H2O molecule to resist. An H+ ion seperates from the H2O molecule to grab the electron. This leaves a negatively charged hydroxl ions (OH(-)) behind with a negative charge that pulls it toward the postive anode. After gobbling up a free electron, the now nuetral H atom quickly reacts with a partner H atom that has also grabbed a free electron to stablize as H2 (hyrdogen gas - ahhh thats what those bubbles on the cathode are!). This is the reduction reaction. The oxidation reaction occurs when negatively charged OH(-) ions reach the anode. Here electrons are pulled from the Ti anode resulting in Ti(4+) cations to be distributed across the surface of the Ti anode. The positive charge of the Ti(4+) cations now act to finish the dismantling of H2O molecules that started at the cathode. Each Ti(4+) ion pulls apart two of the OH(-) anions near the surface of the anode. This results in the release of two more free H(+) ions as well as two O(2-) ions that quickly react with the Ti(4+) ion to form (you guessed it) TiO2.
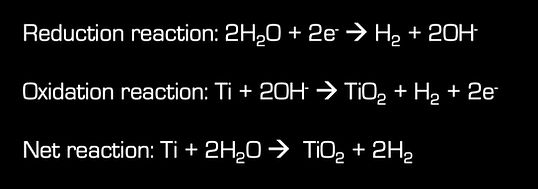
So what's up with the electrolyte? Along with H2O, the electrolyte solution is made up of dissociated ions that provide increased conductivtiy. The addition of these ions to the water is critical to ensure that no voltage drop occurs between the anode and cathode as water alone does not provide a high level of conductivity. But note the lack of the dissociated electrolyte ions involved in the oxidation and reduction reactions. Consequently, anodizing can be accomplished using a variety of different electrolytes such as trisodium phosphate (TSP).
The Color of Ti

Light, TiO2, and the physics of color interference
White light consists of a range of different wavelengths (380-700 nm) that make up the visible light spectrum, which is detectable by the human eye as color. The range of different color wavelenths is best demonstrated by the dispersion of light through a prism, which seperates the wavelengths into a rainbow of different colors (remember that science class?). In most cases, our eyes detect the color of an object due to the presence of pigments or dyes that reflect (typically at the molecular level) a particular wavelength of visible spectrum while absorbing others. The particular wavelength that is reflected is what enters the eye and is interpreted by the brain as the corresponding color. A relavent example of this is anodized aluminum parts. Unlike titanium, anodizing aluminum creates a porous oxide layer that is then dyed to achieve the desired color. But titanium is different...
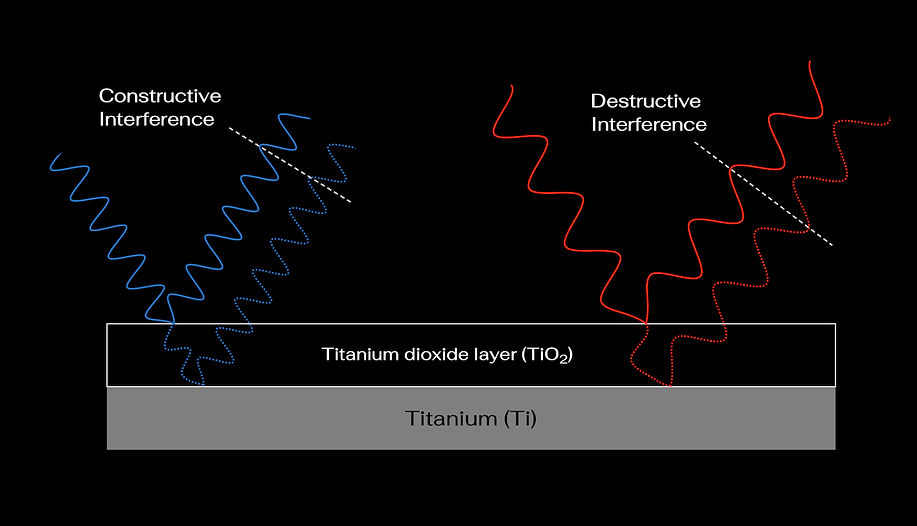
Rather than the surface of an object reflecting a specific color wavelength and absorbing others, the physics of color interference rely on the "thin film effect", which occurs when light waves reflected from the upper and lower boundary of a thin translucent film interfere with one another. This interference reinforces certain wavelengths of the spectrum (constructive interference), while canceling out others (destructive interference). Those wavelengths that are reinforced are what is ultimately the color detected by our eye's. The oil-slick of colors seen on soap bubble is an everyday example of color interference, but guess what else is really thin and translucent? Thats right - the titanium oxide layer!
This is probably easier to visualize, so take a look at the graphic above. For titanium, as the thickness of the TiO2 layer is increased during anodizing between approximately 20-200 nm (it is actually thinner than the wavelengths of the visible light spectrum!), the physics of color interference take hold. When anodized titanium is exposed to light, the translucent TiO2 layer allows some light to pass through it to be reflected off the surface of the underlying Ti metal, while some light is also reflected off the surface of the oxide layer itself. Light reflecting off the underlying Ti surface interferes with light reflected off the suface of the top of the TiO2 layer resulting in a limited range of wavelengths reaching your eye.
​
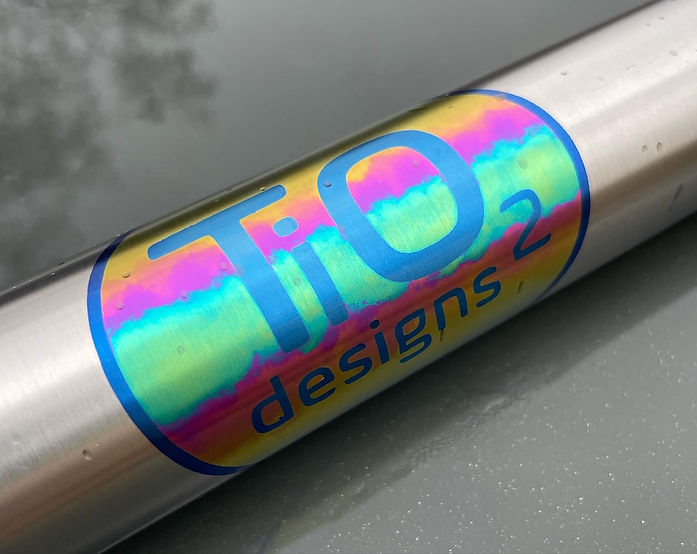